Local Scintillation Measurements
Ionospheric scintillations
Ionospheric scintillation is a phenomenon that occurs in the Earth's ionosphere, which is the layer of the Earth's atmosphere that is ionized by solar radiation. Scintillation refers to the rapid and intermittent fluctuations in the amplitude and phase of radio signals as they travel through the ionosphere.
Ionospheric scintillation can mainly occur in two forms: phase scintillation and amplitude scintillation.
- Phase scintillation occurs when the phase of the radio signal fluctuates rapidly, causing random advancing or delaying of the signal wave front. This can cause errors in navigation and communication systems that rely on precise timing, such as GNSS.
- Amplitude scintillation occurs when the amplitude, or strength, of the radio signal fluctuates rapidly. This can cause fading or dropouts in the received signal, making communication difficult or impossible.
Impact on modern technology
Ionospheric scintillation can have a significant impact on modern technology that relies on radio signals for communication and navigation. Some of the ways in which scintillation can impact modern technology include:
- GNSS: Scintillation can cause errors in the GNSS signal, leading to inaccuracies in position, velocity, and time calculations. This can affect a wide range of applications that rely on GNSS, including navigation, timing, search and rescue services, and remote sensing.
- Communications: Scintillation can cause fading or dropouts in the received signal, making communication difficult or impossible. This can affect satellite-based communication systems, such as satellite phones.
- Remote sensing: Scintillation can affect the quality of data collected by remote sensing systems, such as weather satellites, which rely on radio signals to collect data. This can lead to inaccuracies in weather forecasting and other applications that rely on remote sensing data.
- Aviation: Scintillation can cause errors in navigation systems used by aircraft, which can affect flight safety.
In summary, ionospheric scintillation events can have deleterious impact on communication and navigation. It can cause errors, inaccuracies, and disruptions that can affect a wide range of applications and industries.
Real time scintillation monitoring
Monitoring ionospheric scintillations is important for several reasons:
- Satellite navigation and communication: Ionospheric scintillations can affect the transmission of radio signals used for satellite navigation and communication. This can lead to errors in GNSS location, loss of signal, or even complete signal blackout. This can have a significant impact on transportation, navigation, and communication systems that rely on satellite signals.
- Space weather: Ionospheric scintillations are closely related to space weather phenomena such as solar flares and coronal mass ejections. By monitoring ionospheric scintillations, scientists can better predict and prepare for these events, reducing the potential impact on technology.
- Research and Development: Ionospheric scintillations can provide valuable information about the ionosphere and its dynamics. Understanding these phenomena can help improve our understanding of the Earth's upper atmosphere, which, in turn, can be used to predict scintillations, improve communication and navigation systems, and advance other applications.
- Predictive capability: By monitoring scintillations, scientists and engineers can gain a better understanding of the conditions that cause scintillations and use this information to predict when and where scintillations are likely to occur. This allows them to take measures to protect technology and minimize the impact of scintillations.
- National security: Ionospheric scintillations can also have an impact on military operations that rely on satellite navigation and communication. Monitoring these scintillations can help ensure the continuity of military operations and protect national security.
Therefore, monitoring ionospheric scintillations is crucial for ensuring the reliability and security of satellite-based systems, predicting and mitigating the effects of space weather, advancing scientific knowledge and supporting national security.
The provided provided scintillation indices are observed by high-rate GNSS receivers operated by DLR on all visible GNSS links according to the configuration of the GNSS receivers. The amplitude scintillation index S4 is defined as the standard deviation of the hig-rate (e.g. 50-Hz) raw signal power samples normalized to the average signal power over an interval of 60 seconds. The phase scintillation index σφ is defined as the standard deviation, of 50-Hz detrended carrier phase samples averaged over an interval of 60 seconds. Both of these values are produced as output of the GNSS receivers every minute.
Name | Location | Country | Latitude [°N] | Longitude [°E] | Operator | Equipment |
---|---|---|---|---|---|---|
MSFO01 | Fortaleza | Brasil | -3.74 | -38.58 | DLR / Federal University of Ceará (UFC) | Javad DELTA G3T (50 Hz), Leica AR25 |
MSKI01 | Kiruna | Sweden | 67.84 | 20.41 | DLR / Swedish Institute of Space Physics (IRF) | Javad DELTA G3T (50 Hz), Leica AR25 |
MSNZ02 | Neustrelitz | Germany | 53.33 | 13.07 | DLR | Javad DELTA G3T (50 Hz), Leica AR25 |
MSRF01 | Ramfjordbotn | Norway | 69.58 | 19.22 | DLR / EISCAT | Javad SIGMA G3T (50 Hz), Leica AR25 |
MSST01 | Stanford | USA | 37.43 | -122.17 | DLR / Stanford University | Javad Delta G3TH (20 Hz), Trimble Zephyr Geodetic II |
MSTE01 | Teneriffe | Spain | 28.48 | -16.32 | DLR / Universidad de La Laguna (ULL) | Javad Delta 3 (50 Hz), Trimble Zephyr Geodetic II |
MSTO01 | Toulouse | France | 43.56 | 1.47 | DLR / SUPAERO | Javad Delta G3TH (50 Hz), Topcon CR-G3 |
MSTR01 | Tromsoe | Norway | 69.68 | 18.98 | DLR / Tromso Geophysical Observatory (TGO) | Javad Delta 3 (50 Hz), Leica AR25 |
Daily plots
MSFO01 - Fortaleza, Brasil
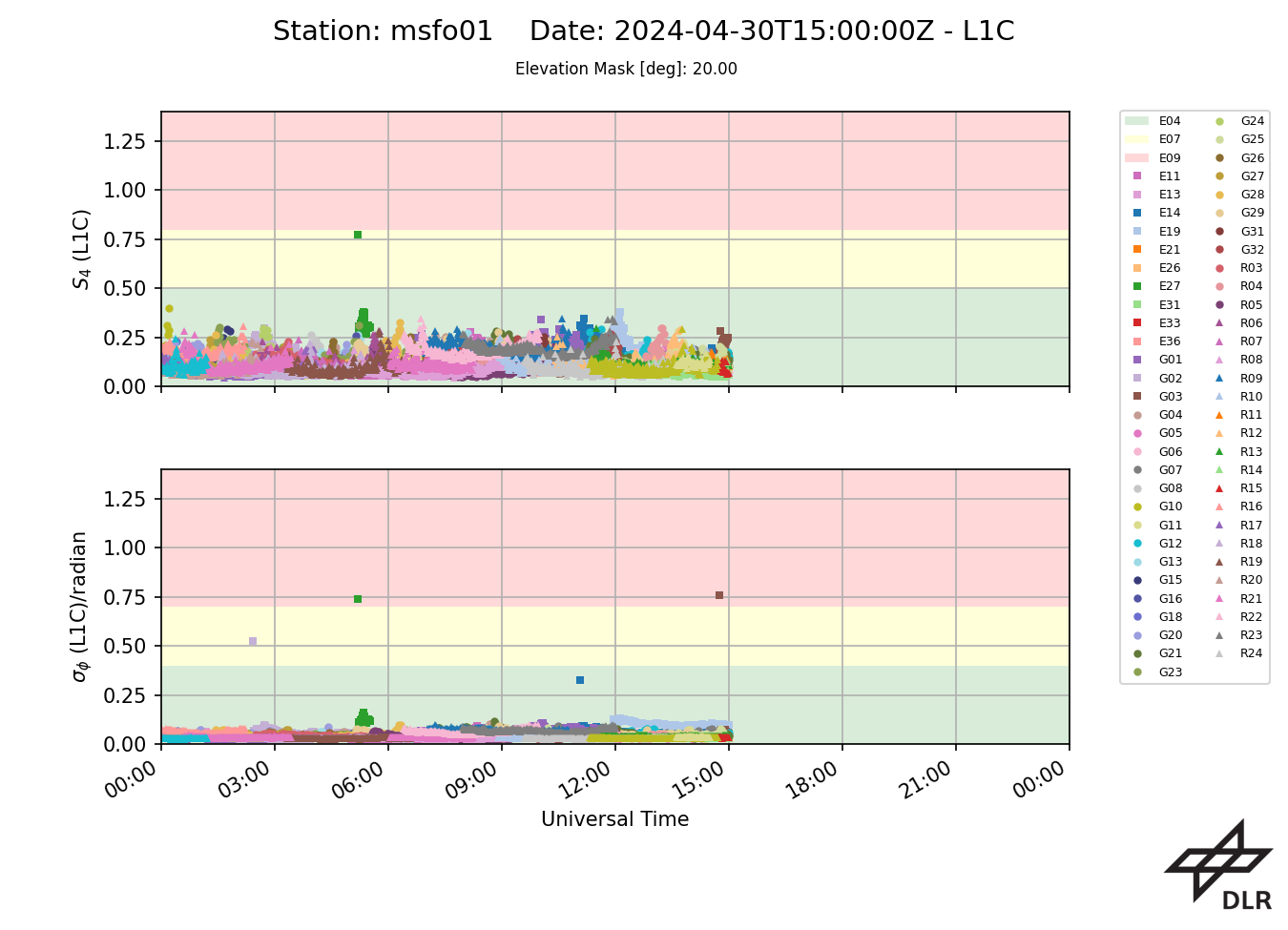
MSKI01 - Kiruna, Sweden
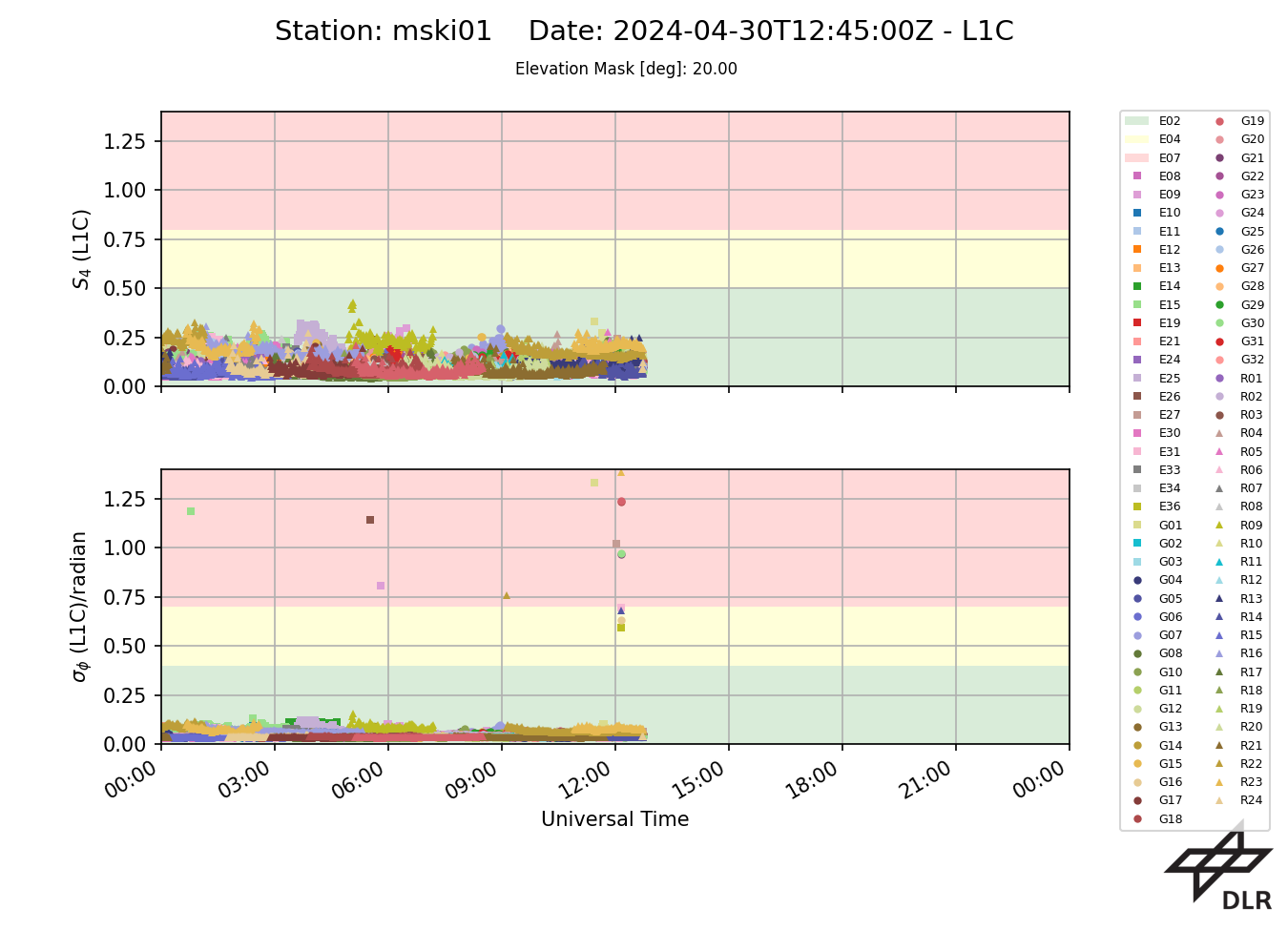
MSNZ02 - Neustrelitz, Germany
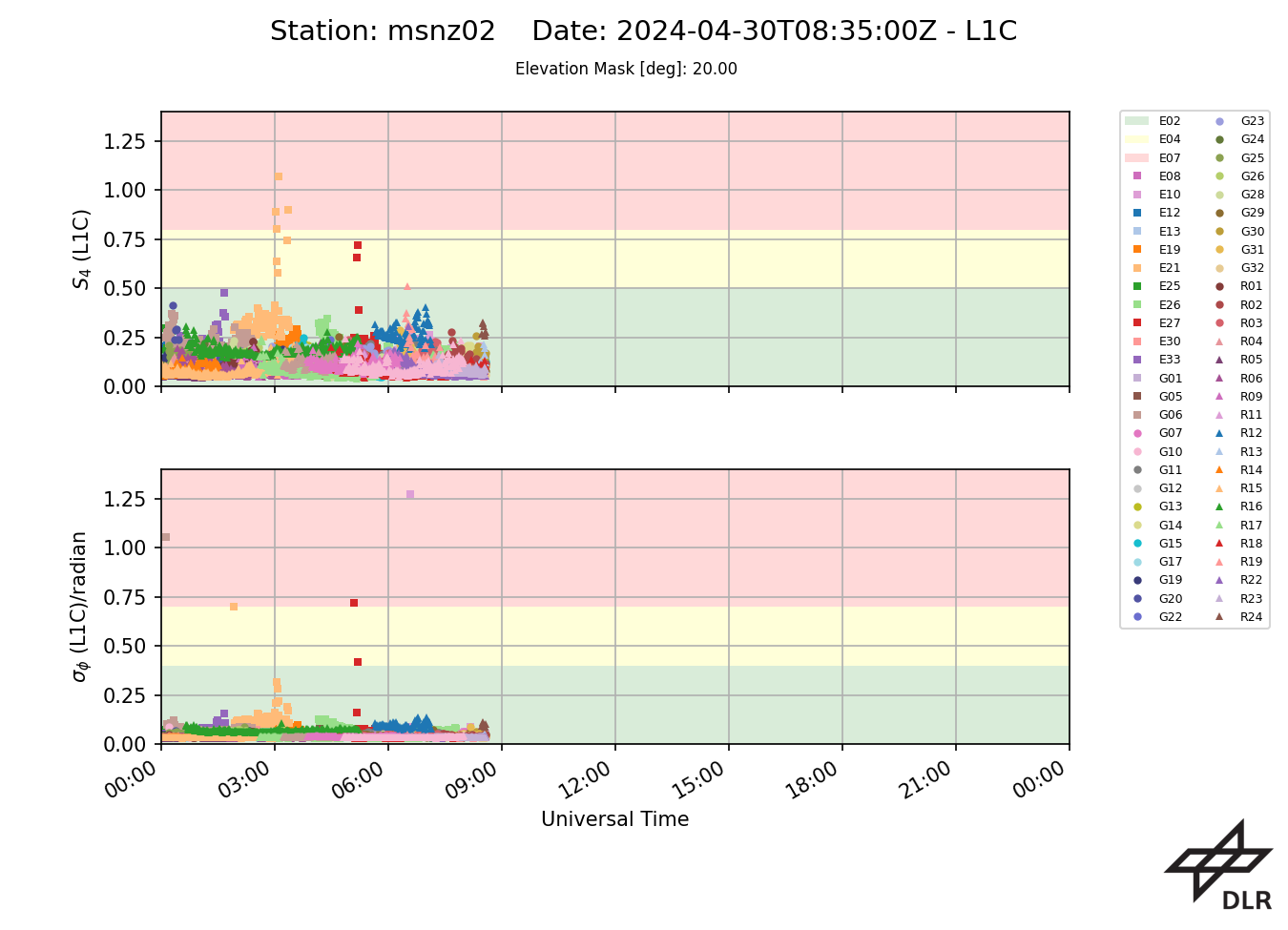
MSRF01- Ramfjordbotn, Norway
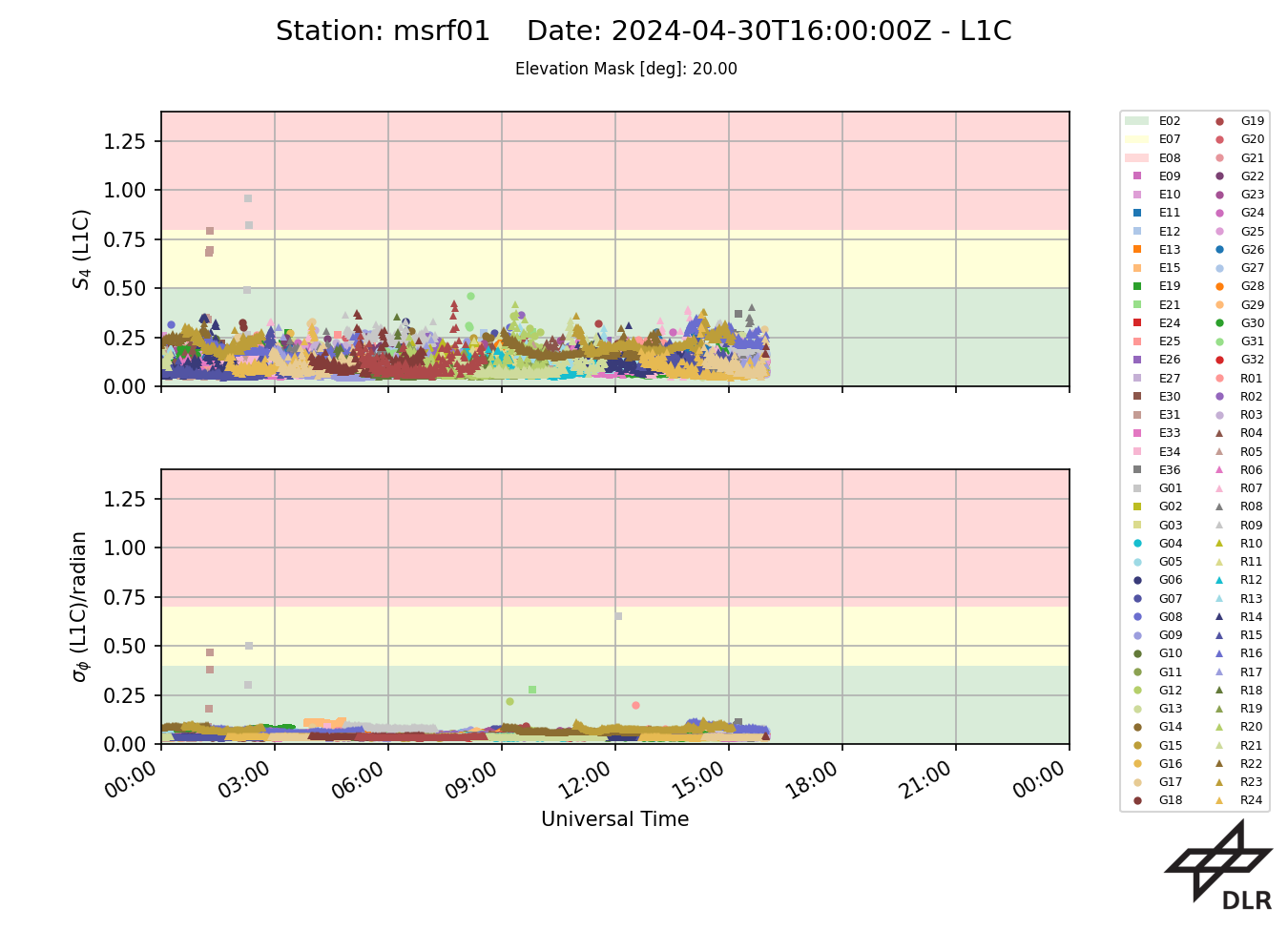
MSST01- Stanford, USA
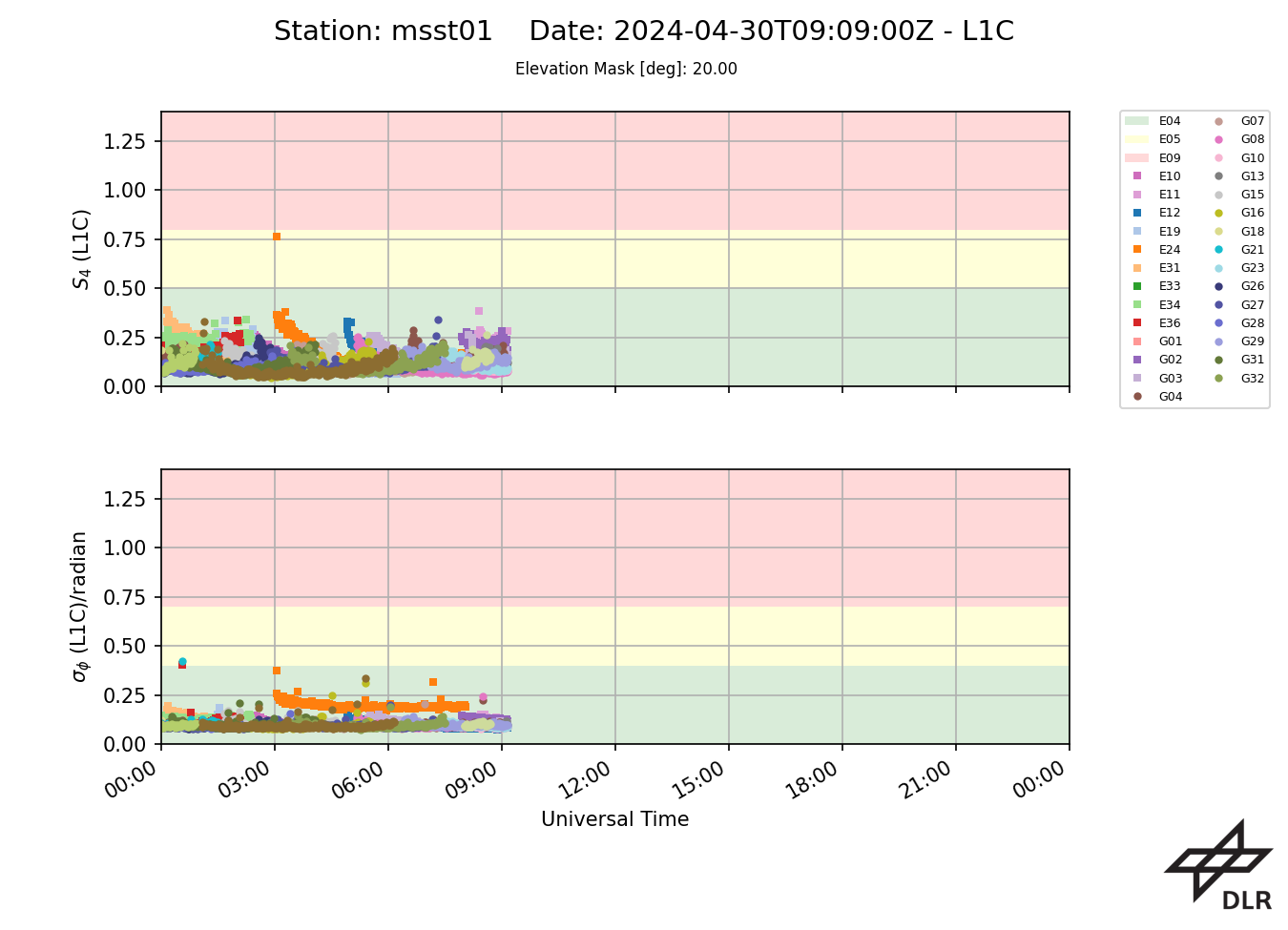
MSTE01- La Laguna, Spain (Teneriffe)
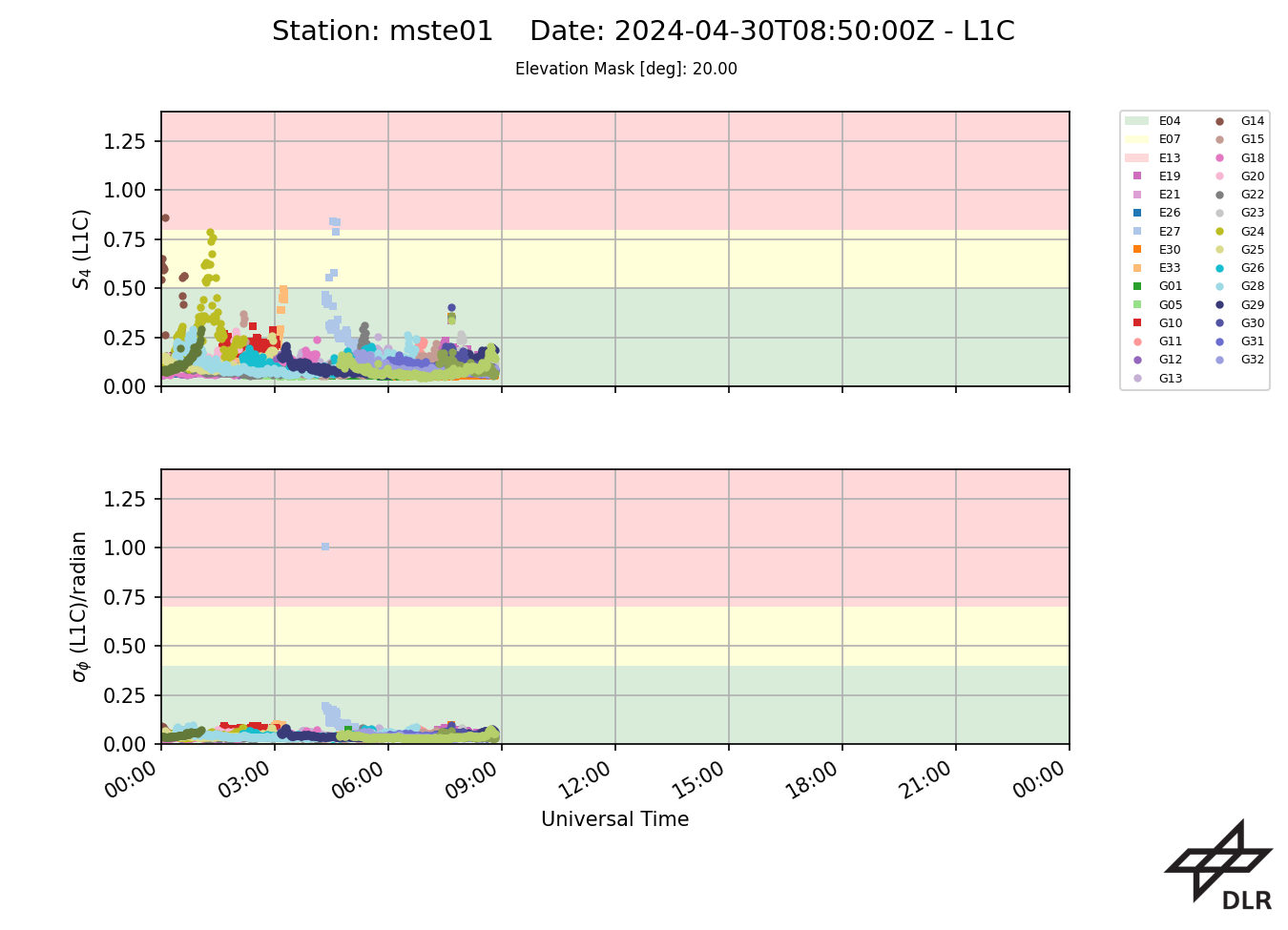
MSTO01 - Toulouse, France
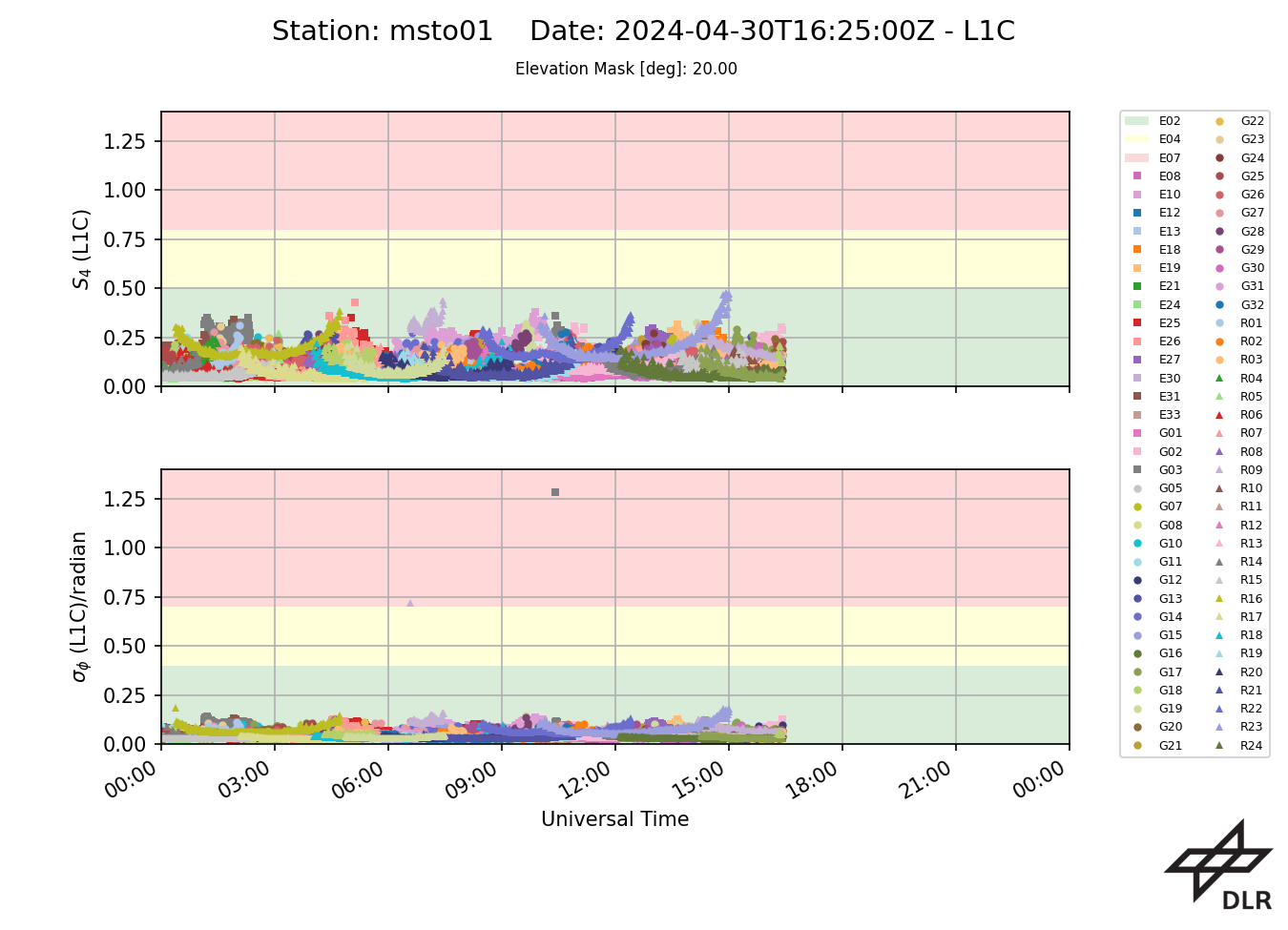
MSTR01 - Tromsoe, Norway
